Fusion Ignition: What Does The NIF’s 1.3 MJ Yield Mean For Fusion Research?
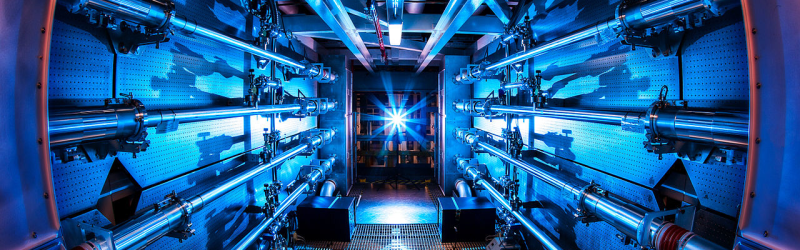
Earlier this month, Lawrence Livermore National Laboratory (LLNL) announced to the world that they had achieved a record 1.3 MJ yield from a fusion experiment at their National Ignition Facility (NIF). Yet what does this mean, exactly? As their press release notes, the main advancement of these results will go towards the US’s nuclear weapons arsenal.
This pertains specifically to the US’s nuclear fusion weapons, which LLNL along with Los Alamos National Laboratory (LANL) and other facilities are involved in the research and maintenance of. This traces back to the NIF’s roots in the 1990s, when the stockpile stewardship program was set up as an alternative to nuclear weapons testing. Much of this research involves examining how today’s nuclear weapons degrade over time, and ways to modernize the existing arsenal.
In light of this, one may wonder what the impact of these experimental findings from the NIF are beyond merely ensuring that the principle of MAD remains intact. To answer that question, we have to take a look at inertial confinement fusion (ICF), which is the technology at the core of the NIF’s experiments.
Everything Is Better With Lasers
ICF is one of the two main branches of fusion research, the other being magnetic confinement fusion (MCF) which includes today’s tokamaks and stellarators. Much like with the initial optimism in MCF and the crushing disappointment when Z-pinch fusion turned out to be unworkable, ICF has had its own share of disappointments. Although it was initially regarded to be a practical way to produce power from fusion, it soon turned out that the power requirements to initiate (ignite) fusion were much higher than estimated, and far less easy to achieve.
MCF found a second life in the form of tokamak and stellarator research, which were more complicated, but promised solving the Z-pinch issues, in particular the plasma instabilities. ICF got a fresh start with the invention of powerful lasers, which might be powerful enough to heat fuel and initiate fusion. This process involves subjecting a sphere of fuel uniformly either directly to laser energy (direct drive), or indirectly (indirect drive).
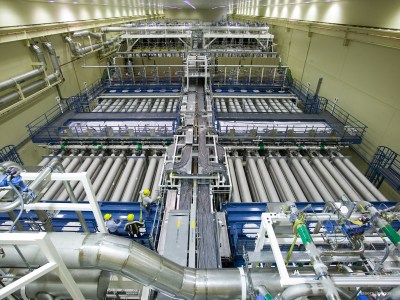
LLNL has been involved in ICF since the 1950s, but only in the 1970s with the advent of more powerful lasers could the first high-power experiments take place. These included the Shiva laser in 1978 and the Nova laser starting in 1984. Both of these laser systems failed to achieve ignition and only got middling results, mostly due to variations in the laser beam’s irradiation.
Despite a funding crunch for fusion research in the 1980s, the findings from these experiments ultimately helped LLNL to establish the NIF, which can be regarded as the successor to the Shiva and Nova laser programs. Through its primary objective of research for the US’s nuclear stewardship program it was ensured of funding, even though construction of the NIF would take significantly longer than originally planned.
Similar to the NIF is the Z Pulsed Power Facility (also known as the Z machine) at the Sandia National Laboratories in New Mexico. This facility uses the Z-pinch principle, making it an MCF system. Despite Z-pinch-based MCF having been discarded as an avenue for power production, it is nevertheless useful for research purposes, making it an important part of the same nuclear stewardship program.
Putting Things Into Perspective
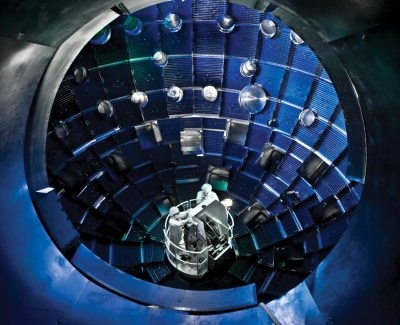
Summarized, the stages in ICF to achieve fusion involve heating up the surface of the fusion target, causing the resulting plasma envelope to expand and thus compressing the fuel. This compression increases the temperature and density of the fuel to the point where it ignites, meaning that the alpha particles produced from fusion are captured inside the fuel and contribute to heating it up.
This in turn causes more fusion events inside the fuel, triggering a chain reaction which ideally fuses all of the fuel, thus releasing all of the potential energy.
In the case of the NIF, a 500 TW (pulse) laser is used, delivering all of the energy to the target within a few picoseconds. Due to the intense stresses on the laser system the NIF is limited to a few hundred firings per year. The fuel is generally not directly irradiated by the laser, but is contained in a Hohlraum, which is a specially shaped hollow object that, when the laser beams enter the cavity (not hitting the fuel pellet) causes it to emit a specific wavelength of radiation. In the case of the NIF this Hohlraum is designed to produce X-rays. This Hohlraum can have two entrance holes, or more, as detailed for e.g. a three-axis hohlraum design by Longyu Kuang et al.
These X-rays heat up the fuel pellet, starting the fusion process. The advantage of using a Hohlraum is that it makes an even heating of the fuel pellet much more straightforward. Even so, the energy requirements to heat up the fuel pellet with ICF are immense. This is detailed in the following diagram from the Wikipedia entry on the NIF:
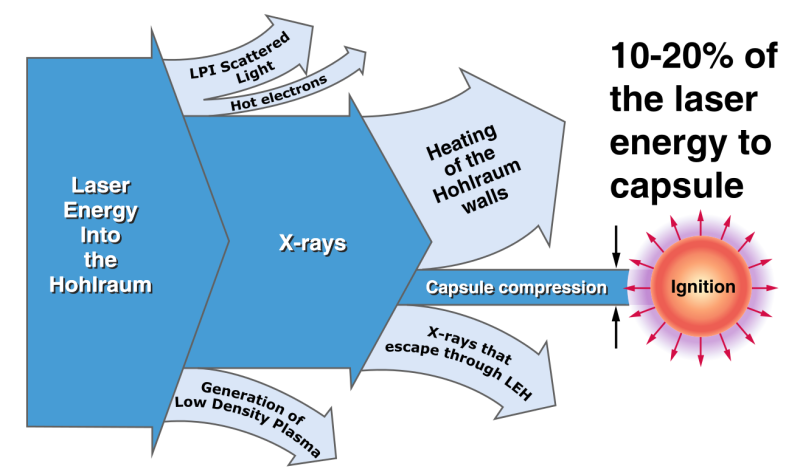
It takes roughly 422 MJ of energy to power the laser system (total system input), of which only a percentage is converted into the laser beam energy that ultimately makes it to the Hohlraum. Of this energy, most contributes to the generating of X-rays within the Hohlraum, only a fraction of which ultimately contributes to the compression of the fuel pellet.
When one considers that it took 422 MJ in order to get a return of approximately 1.3 MJ out of the fuel target, it probably does not require an extensive explanation why this approach to fusion is unlikely to ever reach the point of breakeven, i.e. a Q of 1. The notable aspect here is rather that this amount of released energy was 70% of the input laser energy, meaning that ignition was almost achieved.
No More LIFE
Between 2008 and 2013 LLNL did in fact work on the Laser Inertial Fusion Energy (LIFE) effort which sought to turn the lessons from NIF into a nuclear fusion power plant, using solid-state semiconductor lasers and mass-produced fuel. Yet by the end of the project’s life it was clear that a power plant using ICF was eminently unrealistic, especially in the light of ICF ignition not having been achieved yet.
When one compares the monumental challenges that ICF faces in competing with MCF approaches, with the JET and HL-2M tokamaks as well as the Wendelstein-7X stellarator showing highly promising results in terms of plasma stability and heating, not to mention a Q factor that is tantalizingly close to 1, it should be clear that ICF is not a player in the future power plant game, barring some amazing breakthrough.
What ICF projects like the NIF do however contribute to is increasing our understanding of physics, not just nuclear physics, but also those related to exceedingly powerful laser systems and energy physics in general. That by itself makes this almost-ignition event at the NIF something to cheer at.
[Heading image: Preamplifier at the National Ignition Facility by Lawrence Livermore National Laboratory CC-BY-SA 3.0]
from Blog – Hackaday https://ift.tt/3zH4hhi
Comments
Post a Comment